Calico’s Cynthia Kenyon on The Science of Aging
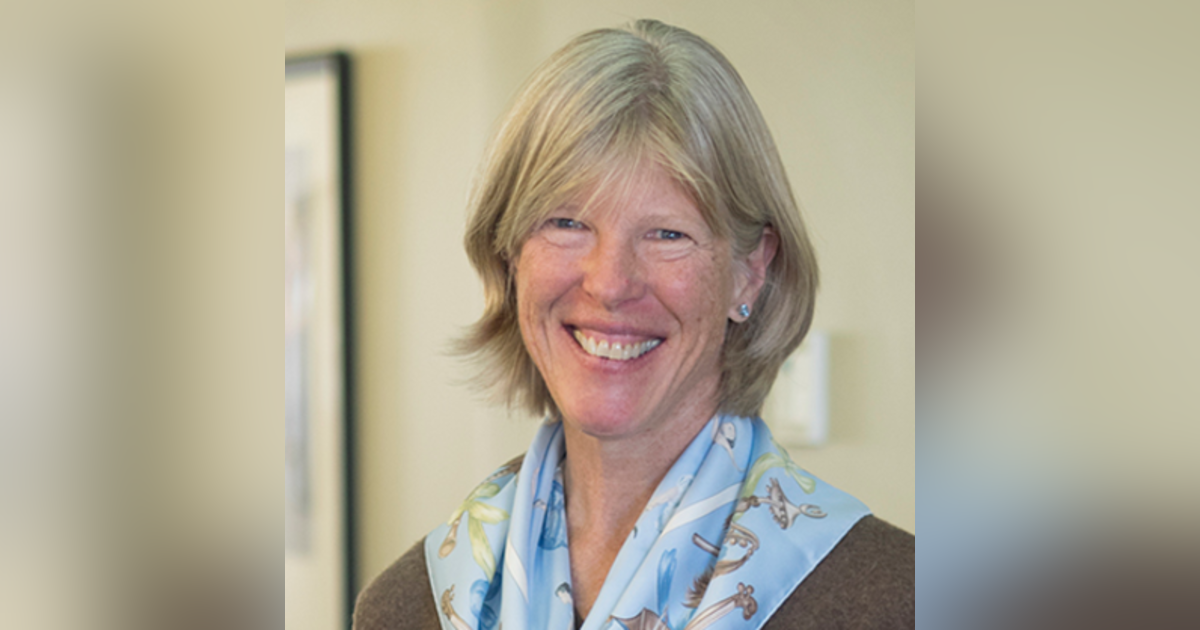
For millennia, humans have believed that aging is inevitable. Yet thirty years ago, the work of Professor Cynthia Kenyon and her colleagues showed that a single gene mutation in a worm doubled its lifespan and postponed the diseases of aging. Recent work on the naked mole rat, a mammal like us, has shown that risk of death need not increase with age.
In the final episode of this season of Theory and Practice, we explore the genetic, cellular, and molecular basis of aging with Professor Kenyon and ask what harnessing this knowledge means for the future of healthcare.
Theory and Practice is a presentation of GV and Google AI.
This season we'll dive deep into the languages of life through explorations of the "dark genome", genome editing, protein folding, the future of aging, and more.
Hosted by Anthony Philippakis (Venture Partner at GV) and Alex Wiltschko (Staff Research Scientist with Google AI), Theory and Practice opens the doors to the cutting edge of biology and computer science through conversations with leaders in the field.
For millennia, humans have believed that aging is inevitable. Yet thirty years ago, the work of Professor Cynthia Kenyon and her colleagues showed that a single gene mutation in a worm doubled its lifespan and postponed the diseases of aging. Recent work on the naked mole rat, a mammal like us, has shown that risk of death need not increase with age.
In the final episode of this season of Theory and Practice, we explore the genetic, cellular, and molecular basis of aging with Professor Kenyon and ask what harnessing this knowledge means for the future of healthcare.
Theory and Practice is a presentation of GV and Google AI.
This season we'll dive deep into the languages of life through explorations of the "dark genome", genome editing, protein folding, the future of aging, and more.
Hosted by Anthony Philippakis (Venture Partner at GV) and Alex Wiltschko (Staff Research Scientist with Google AI), Theory and Practice opens the doors to the cutting edge of biology and computer science through conversations with leaders in the field.
Anthony 00:06
Hello, you're listening to the third series of Theory & Practice, all about science that will be most impactful in the future. I'm Anthony Philippakis.
Alex 00:14
And I'm Alex Wiltschko.
Anthony 00:16
Today's episode is the last of this series. And what better way to finish off than exploring our own ends as human beings. Our topic today is the future of aging. We'll explore: how long will we live and how long can we live.
Alex 00:31
All the way back to the Greeks: we thought that life just has an end, death for humans is as inevitable as a fire left to burn.
Anthony 00:42
This view that life can't be extended has persisted for over 2000 years. Ask most people if they think your chance of dying increases as you age, and they'll say, “Well, duh!” Intuitively, they're expounding Gompertz law. In 1825, the mathematician spotted that deaths seem to follow a geometric progression. The older you are, the greater your chance of dying. Makeham later added a correction for unnaturally early deaths from war and famine. This formula of increasingly diminishing lifespan remained unchallenged until 1993.
Alex 01:17
Nearly 30 years ago, one scientist turned all this on its head with the discovery that a single gene mutation could double the lifespan of a worm called C. elegans. Not only were these worms with the mutation long lived, they also ducked many of the diseases of aging. So this is all very exciting for worms. But what is it got to do with us? We're complex mammals. Enter the naked mole rat. This incredibly long-lived, pale, fetal-like kind of gross looking mammal lives five times longer than expected for its size. A paper in 2018 showed that it totally confounded Gompertz’s law, there was no increase in its risk of death as it got older. This was a non-aging mammal.
Anthony 02:05
Today, our guest is the amazing scientist who overturned the existing paradigms of aging. Professor Cynthia Kenyon is the Vice President of Aging Research at Calico Life Sciences, and emeritus Professor of Biochemistry and Biophysics at the University of California San Francisco. With her, we are going to explore just what is known about aging, and what this means for the future.
Professor Cynthia Kenyon: Welcome to Theory & Practice.
Cynthia 02:44
Thank you so much.
Anthony 02:46
So let's dive straight in. What happens to our bodies when we age?
Cynthia 02:50
Well, there's a general physiological decline in many different abilities, many different systems. Your musculature, your grip strength, how fast you can run declines, how much air you can expel from your lung declines. Your ability to regulate your metabolism declines. Many people, when they're young, can eat a lot of sweets, and it doesn't matter, they don't get fat, they're fine. But when they get older, it's not the same way, and their blood pressure goes up. So it's a kind of broad systems decline. But it's very characteristic. For example, if you look at an old dog and an old human, you could tell right off the bat that they're both old. And almost all old animals share some obvious feature, a je ne sais quoi. You can't really put your finger on it. But you know that's old, it's not sick like a motorcycle victim in an accident. It's not that it's a particular kind of decline, that has a very universal manifestation across all of biology.
Anthony 03:52
Let's go one level deeper at the level of our cells, or at the level of our genome, what's happening as we age.
Cynthia 03:59
Again, there's a general decline, for example, the pH of the lysosome. It's a bit of the pantry of the cell. And it also is important in protein degradation and turnover. It's normally acidic. It is acidic when you're young. But as you get older, the pH rises a little bit so it doesn't work as well. The mitochondria don't work as well; in many ways, mitochondrial DNA tend to suffer mutations. They're just not as good at making ATP and running the Krebs cycle and doing the things that mitochondria are supposed to do. Also, the mitochondria can leak a little bit. Damaged DNA, for example, can get out of the mitochondria into the cytoplasm. And this and other changes can cause inflammation to increase. And this is also a very characteristic hallmark of aging. It can arise from damage in the mitochondria, but it can have other origins as well. You asked about the nucleus, other changes in heterochromatin in DNA methylation, which changes gene expression also.
Alex 04:56
Sounds like changes across the board and kind of a decline in the performance or the output of different parts of our body. How do you model that? What are the main ways of actually studying this decline closely?
Cynthia 05:10
Well, one thing you can do is you can study lifespan. That's very crude. But the rate of aging is absolutely connected to lifespan almost all the time. You could also measure what you call a health span, which you can measure at the level of physiology, you can measure grip strength decline, you can measure the ability to expel air from the lungs, things like that, that take place at the level of physiology, you can zoom down and you can study lysosomal pH, if you want to. You can really set up an assay for almost any aspect of aging that you want to. And what often people do is they'll look at more than one hallmark of aging. So they'll look at cellular features. And you'll look also in addition at organismal physiological features.
Anthony 05:55
One of the things that's so interesting is we're starting to see the rise of unifying models in what the process of aging is. Can you overview for us some of these models of aging? And what evidence there is for them?
Cynthia 06:08
Well, first of all, we know from genetic studies something that we didn't really know, although we should have been able to infer just by looking around at animals with different lifespans, which is that genes control the rate of aging. And one way they do that is by setting resilience levels in the cell. And that involves proteostasis capacity and DNA repair activities. You know, resilience and repair activities are set at different levels in different organisms. And that, then, can influence the rate of aging. So that's one thing that isn't obvious just from looking at aging itself, but it definitely underlies it. And it was something that came from genetic studies; it wasn't something people intuited. There are many things that change with age, and very interestingly, and surprisingly, if you fix any one of them, or many of them, you can have really big beneficial effects on mouse lifespan, for example. So, telomeres shorten with age, and that causes cells to become senescent and it causes other aspects of decline. There are two reports now, saying that if you extend telomeres or you prevent the shortening in mice, you extend their lifespan.
Anthony 07:19
I remember when I first entered med school, there was a lot of speculation that it was the accumulation of damage to the genome. More recently, we've seen the rise of cellular senescence as being a potential cause of aging. What do you think of that idea?
Cynthia 07:32
Well, senescent cells accumulate with age. If you clear senescent cells, there's a small effect on maximum lifespan but a very large effect on healthspan on the rate of aging. There are factors that circulate in the serum whose levels change with age. If you take the blood from a young animal, and you introduce it into an older animal, you can slow down aging. Now these might be related in some way; it's not really clear. There are changes in, for example, the way cells polarize themselves. Stem cells divide asymmetrically to make a copy of themselves generally, and some other kinds of cells that differentiates into a specialized cell type, like a muscle cell, for example. And the ability of cells to remain polarized changes during aging. And apparently, if you make changes in animals that would be predicted to repolarize cells, the mice live long. So it's strange, because what happens when you make one change? Does everything else get better? We don't really know that. And it's not clear to me that there is a unified theory of aging- I don't know of one. In fact, a new paper came out that's very interesting, suggesting that a primary cause may be the nuclear lamina. You know, that may be the ability of parts of the genome to associate or not appropriately with a nuclear lamina and to enter a state of facultative, or constitutive heterochromatin - to put that into simple language, the ability of the nucleus to arrange the DNA properly - may change with age, and that may give rise to a lot of inflammation. So I'm a scientist interested in aging, and I keep all those theories in my head. Here's another one: the ability of the lysosome to take up substrates that it should degrade, changes with age. There's something called chaperone-mediated autophagy, that appears to change with age, and if you rectify that, then the lysosomes reform better, and mice live longer. So there you go. To me, that doesn't sound like a unified theory; however, it happens, and you have to just keep a lot of possibilities in your mind.
Alex 09:41
Taking a step back, it sounds like a lot of these mechanisms of aging are under the control of our genome, or maybe how the genome is actually physically packed or accessed. But your work is mainly on worms, although you've spoken a lot about mice, and I'm thinking about aging for people, as I'm listening to all this. So how can we learn about our aging from worms?
Cynthia 10:05
Well, C. elegans is the worm you're talking about. And it's a tiny little animal with only about 1000 somatic cells in its body; it's about the size of a comma. And it has a relatively simple genome. But it has pretty much all the main cell types that we have. It's not as sophisticated as a human or a mouse, but it can do a lot. And the nice thing about them for studies of aging is that they age really quickly; they age and die in just a few weeks. So it's also very easy to look for mutations that affect aging or to do either random mutagenesis or to just scan genes one by one, using other technologies like RNAi. So you can identify genes. And once you find a gene that affects aging, you can figure out what it does pretty quickly. And then you can check to see whether what you found is evolutionarily conserved by changing it in flies in mice. And it’s really remarkable, I think, everything…I shouldn't say everything, never say everything…but nearly at least everything that has been found to affect aging, in C. elegans, the worm also affects aging in flies and mice when it's been tested. So it's very, very predictive of higher organisms. Now, there are things that change in mice that don't even happen in worms, like changes in senescent cell abundance - that’s not known to happen in worms. If it does, for example, DNA methylation isn't a big driver in Worms, but still, a lot of things are the same. So we work on worms, but in a way, I think we work on biology, we're working on words.
Alex 11:39
There's another organism that I want to ask you about, which is different in terms of how it ages or this is what I've read, which is the naked mole rat. Could you maybe tell us a bit about the naked mole rat and why it's so interesting for studying senescence and healthspan and lifespan.
Cynthia 11:55
Yeah, it's really interesting. So naked mole rats are about the size of a mouse, but they look a little bit like a burrito with legs. They also look like newborn mice, actually, because they're naked. They don't have hair. They're blind.
Alex 12:08
They're wrinkly and pink and stuff.
Cynthia 12:10
Yeah, but they become mouse-sized. And then they live for at least 30 years - they can. And they don't seem to age during that period.,
Alex
30 years of life?
Cynthia
At least! There are a lot of 30 year old naked mole rats.
Alex 12:25
Wow. And mice live for just a couple of years. Right?
Cynthia 12:29
That’s right. Mice live for about two, on average. Yeah, it's amazing. So this was something that was discovered by Shelley Buffenstein and Graham Ruby at Calico; the mortality rate doesn't increase with age. In other words, a naked mole rat, when it's five years old, has the same chance of waking up in the morning and dying that day, as it does if it's 25 years old. And physiologically, they also don't seem to age when you measure any of a number of different physiological properties that decline with age in mice or people. It's the same in young and old animals.
Alex
So why is that?
Cynthia
It's not clear - it's not clear at all. So I told you that there are resilient systems that govern the rate of aging in animals. The most famous one is the one that my lab helped to discover: the insulin IGF-1 signaling system. That system controls growth, and it controls nutrient utilization. But it also controls resilience, stress resistance. And if you change it in such a way that you change the basal set rate of resilience of the animal, it will live longer. So in mice - you have normal mice, and then you have mice that have been changed by this. And they have a lot of different phenotypes, a lot of different traits that are changed. So their physiology has changed, their muscle to fat ratio has changed, their gene expression profile changes, these naked mole rats have a lot of the same changes that we see in the long-lived mutants. So we haven't been able to go in and change the genetics of a naked mole rat to prove that. But it's very remarkably reminiscent of the changes that occur when you change resilience, suggesting that their resilience mechanisms are just set at a much higher level. There's another thing that's really interesting about them, which is that in many ways, they seem to retain baby traits, traits of very young animals. It looks as though they may not really make it to adulthood ever. So they may be kind of stopped in an adolescent state, like Peter Pan forever.
Anthony 14:30
Now, one of the things you brought up was the insulin signaling and its role in resilience. I mean, that was one of your earliest discoveries was the role of metabolism in aging. So tell us more about the connection between metabolism and aging?
Cynthia 14:46
Well, all animals apparently - we don't know about humans, maybe humans, but - as far as we know, all animals have a way of living longer than they normally do, which is remarkable. And it was a discovery that was first made in C. elegans, if you change this gene, the gene that encodes the receptor for the insulin and IGF-1, hormones, and these are hormones that control the rate of growth, and they control food nutrient utilization, then the animals can have twice as long as normal, and they age much more slowly, but all sorts of criteria. And the reason that happens is because they activate a lot of protective transcription factors - gene regulators - and those transcription factors encode proteins that allow cells to better withstand stress and to repair damage in many, many different ways. So here, you're a worm, you're living your short life, but you have the ability to live a longer life, but you're not using that ability, why not? And the reason is that the stress capacity, the stress response, or resilience capacity that the worm has, is kind of inducible. It can, it could be expressed under certain conditions. So for example, if you subject C. elegans to…if you give it less food to eat, than you would normally give it…If you do that in certain ways, then the animals live longer, because it will activate this resilience mechanism. If you subject it to other kinds of stress, it will activate this mechanism, the mutations that we made, essentially allowing it to think it's under stress, speaking anthropomorphically, even though it's not, and that allows it to live longer. And the same thing is true in flies. The same thing is true in mice. There's many, many, many different ways that you can activate this resilience pathway in mice by changing the same kinds of regulators - insulin and IGF-1 receptors or hormones or downstream signaling components.
Anthony 16:40
So Cynthia, that was an incredible description of the connection between metabolism and aging. You mentioned that it's not clear whether or not it works in humans. You mentioned that in almost every organism, caloric restriction seems to extend lifespan. But you know, that hasn't yet been validated in human beings. Certainly there are people trying to do that experiment right now. What's your take on it?
Cynthia 17:04
Well, first of all, it has been tested in primates; it's been tested in monkeys. And it seems to improve the health of monkeys. But it didn't really improve their lifespan. There were two studies done and at least in one that didn't really appear to extend their lifespan. With humans, there is a caloric restriction society. And there are various different studies that people do. Again, they're destined to be health benefits, particularly in any kind of health parameter that is associated with something like diabetes, and just general energy levels and things like that. There is a lot of, I think, some improvement in that area. But we don't really know about lifespan.
Alex 17:44
So when you were describing your discovery, the link between IGF-1 and lifespan, it reminds me of some of these other protective mutations that Anthony's brought up in previous episodes, like PCSK9, and the fact that that gene doesn't seem to do anything other than make our lives worse. So in that light, would you say that aging is a disease? Should we think of it that way? Or should we think of it in another way?
Cynthia 18:08
Well, I am kind of morally opposed to telling anybody of how they should think, so basically, there's a definition for aging and a definition for disease. And this is kind of an interesting story. Aging is a decline that happens to everyone. A disease is a problem, something that you don't want to have happen to you, that doesn't happen to everyone. And here's why I think that distinction is so interesting; there is a condition called progeria, or getting old faster than normal. And some people have this condition - there are various different progerias. And those are called diseases, even though we're talking about aging. So these people are aging more quickly than normal. But in that case, aging is a disease, because it doesn't happen to everyone. On the other hand, if you had a screen… if let's suppose you're a doctor, and you're taking all these measurements of grip strength, running, all these things, and you don't know how old the people are, and but one person is 85, and one person is 25, you might say one is sick. So you might say it, but because it happens to everyone, we call it aging. So I think it's a little bit semantic.
Anthony 19:17
I think that's a really interesting insight. And maybe what that suggests to me, is that we're asking the wrong question. And instead of thinking about aging as a disease, we should be thinking about extending youth rather than extending life. What do you think of that idea?
Cynthia 19:35
I actually think that's what we want to do. I mean, for me, and for most people in the field, what you really want is to have each day that you're alive, be as good as it can be. And part of that is how your body feels. How quickly can you think, can you remember names? Can you run, can you lift something that used to be able to lift, if that's what you want? You want to be able to do that for as long as possible. I think that's what people in the field are striving for. Lifespan itself…I mean, first of all, it's kind of interesting. But we've made huge increases in the human lifespan, not by studying aging, but by, you know, discovering antibiotics and learning how to cure a lot of diseases. We've added lots of time to people's lives. And a lot of it is good time, really good time. But some of it might be not optimal, you know, people might be alive, but maybe not as healthy as they were once or could be. So I think the idea that part of aging is really a decline of what we call health - health being just the ability to be how you were when you were younger, essentially. So I'm talking in circles here, recursively. But that's really what we're talking about. So I think that the idea of extending what we call the health span is a really wonderful idea that I think could be great for people.
Anthony 20:54
Well, what do you think is the most viable strategy to do that?
Cynthia 20:57
I don't know. I think we should try everything we can. Honestly, I really do. You could imagine that we're all, what I would call, DAF-2 mutants. So remember, I told you that in the worm, and C. elegans, you can change this gene and you activate this resiliency, and they live twice as long. Maybe we've already activated all our resiliency, maybe we've hit our limit, maybe we can never go any further. I don't see why not. But we don't know that. But we know, if you're a mouse these days, you're really lucky because there must be 30 ways that people can intervene - a lot of them are different - and extend their healthspan and lifespan. Right now, the nice thing is that most of…this is a really wonderful, wonderful thing I'm about to tell you…which is that most of the perturbations that you subjected an animal to changes something in an animal that extends their lifespan - most of them have beneficial effects on diseases. And it kind of makes sense, because for some reason being old, means you're more susceptible to diseases. So from a practical perspective…but I'll just use an example. One of my favorite ones is MYC - one of my all time favorite examples. MYC is a huge driver of cancer; John Sedivy’s lab showed - and also it's been shown in worms and flies - he showed it in mice - that if you lower the level of MYC activity in animals that don't have cancer, you'll increase their lifespan. So you can imagine if you had an MYC inhibitor, get a drug like that approved for cancer, and then people will start taking it. And you could ask, if you measure features that have to do with aging? Are they occurring more slowly? And maybe not. But maybe so; maybe you could try 15 or 20 of these different perturbations each for a disease. And while the people are taking these drugs for the disease, assuming they're not too sick, you can look at parameters that more generally affect aging, and then pretty soon, maybe you could start doing aging trials.
Anthony 22:52
Well, correct me if I'm wrong, but something like this is kind of going on right now with Metformin.
Cynthia 22:56
Yes. So Metformin is a very safe compound that you can take that's very beneficial for Type 2 diabetes. And most people that have Type 2 diabetes, take Metformin. And it was found that people who take Metformin have a lower chance of getting another disease, than do ordinary people who don't even have diabetes, which is remarkable. One of the traits of aging, besides just this physiological decline is an increased chance of getting diseases. So if your chance of getting any age related disease goes down, well, that could be a sign that you're aging more slowly. So you can imagine a clinical trial, where you take a person who's had one disease, those people are more likely to have another, give them Metformin and see if the chance of getting any other disease goes down. And there is a trial called the TAME trial (Targeting Aging with Metformin), which is designed by a consortium - Nir Barzilai and others - that's designed to measure the effectiveness of Metformin, not just on diabetes, but on all age related diseases. And that's an interesting trial design.
Alex 24:02
So don't treat one disease, treat all of them at once. And see, kind of see what happens.
Cynthia 24:08
That's the real promise of aging: it's like magic, you could have effectiveness against all diseases, and your health could decline more slowly, it would be amazing.
Alex 24:17
The scale of that is really staggering. I mean, there's some diseases or some scientific problems where you could imagine just retasking all intellectual activity on Earth and solving the problem in five years. So like, let's say, we took every biologist and said, you're working on the Dark Genome, right? Maybe, you know, I mean, there's a fundamental limit to how fast we can progress our knowledge, but you can imagine trying to accelerate that progress really, really fast. There's something different about aging research, especially in humans, that makes the project fundamentally last multiple lifetimes. I mean, every shot on goal is a human life in a way. How do you think about the scale? There's the almost requisite scale of this research programme?
Cynthia 25:00
Well, first of all, you don't have to measure lifespan to measure aging. And there's all sorts of different ways that you can do it, I just told you about one, looking at the chance that you'll get an age-related disease. But there are a lot of other ones: physiological changes, frailty, methylation clocks, you know, or a combination of these things. There's a lot of ways that you can imagine measuring this decline in the rate of decline changes, on average, with a certain value, let's say over five years, you could imagine doing a trial and measuring all these different indices: blood pressure, grip strength, whatever, walking speed, or combination, along with maybe some more molecular phenotypes, and then you could learn probably in five years or less, even whether the rate of change has declined. And if something isn't harmful, at the rate of changes decline, especially if the same drug can be used for some disease, you know, makes it very, very plausible.
Alex 25:58
Could you maybe tell us, what we should be looking for in the future? Maybe the near future, maybe the long term in terms of what is on the horizon? For therapeutics here, you told us about Metformin. But what else should we be listening for looking for?
Cynthia 26:12
There are a lot of different age companies that are studying, trying to intervene to slow down aging, there are at least 100. And they're using very different approaches. In fact, I was telling you earlier, that there's more than one way to slow down aging in a mouse. Well, if you just list all those ways of slowing aging down in a mouse, most of those ways are currently being investigated in biotech companies or in academia to try to find small molecules or antibodies or something that you could give people to mimic that effect. So it's going on right now. I've always felt for a long time that it's really a shame that the National Institutes of Health has such a small budget for aging research. It is tiny compared to cancer, or Alzheimer's disease or any of the specific diseases. And I think part of that is because we just think aging always just happens. It's not a disease. But we might think that aging at this rate has to happen, because everybody does it. So the concept that it doesn't have to is not really…people still don't really get it, I think… that as a concept. We don't know, but as a consequence, the National Institutes of Aging budget is tiny, it's hard to do a mouse experiment in academic labs, because it costs too much money. So that's a problem. There are lots of companies that are well funded now. But we'll see. You know, once it happens, once we really slow down aging, I think everything will change.
Anthony 27:34
Well, you know, I think one of the reasons why aging doesn't get the funding that it deserves, is that when people first hear about the idea of working on aging, and trying to slow it down, it often sounds impossible. And people first encountered it and felt skepticism. But in many ways - and I don't want to embarrass you - you were really one of the first scientists to ever show that it could be done in your work on IGF that we've talked about earlier. I have to believe that when you were first starting out in this field, you must have encountered an incredible amount of skepticism and people really pushing hard against the idea that this was possible. Tell us a little bit more about your own personal resilience, not just cellular resilience.
Cynthia 28:22
Yeah, it was really, really hard to get the project going. I was a professor at UCSF, and I had done important studies in developmental biology and C. elegans. And when I started talking about studying aging, looking for long-lived mutants, everybody had a reason why you couldn't find them. And not only that, there is kind of a little bit of a social hierarchy and maybe snobbishness in science. And if you start talking about something that sounds crazy, people don't just tell you that it's unlikely to work, they kind of sneer at you, they kind of treat you as though you're a stupid idiot, basically. Even if you've done good work in the past that's acceptable and kind of mainstream. So it was really hard. I mean, one person told me that they knew other people who tried to study aging, and they would fall off the edge of the world. It's as though the world really was flat, and you thought it was round, and off you went. These are all people in the National Academy, by the way. Another person told me that you could never get long-lived worms because they had a stereotype cell lineage, so it wouldn't be possible, which is crazy, but that's what they said. They all have these reasons. But the fact is, it's not just me; the first long-live C. elegans mutant was discovered by other people. Nothing much was known about it, but it was known that there was a mutant that live longer, didn't reproduce very well, but it was out there. And it was possible to follow that through to learn what the molecular function was. We've discovered two genes actually, one that was when you inhibited it, the worms live longer, and one that was needed for that long lifespan. And that one, the second one was a transcription factor. It'll be cloned it in our lab and showed that. So that showed right away that there's a gene regulatory program for aging, which is amazing. It was a big deal. So I think the other thing was we know that transcription factors are, we know what hormones are, we know it receptors are, AKG signaling pathways. People know what that is. And they think it's serious science. So I think it really put aging…you couldn't just say that aging was some kind of non science anymore. It was real science controlled by very well understood molecular mechanisms.
Anthony 30:31
What motivated you to even begin to work on aging in the first place?
Cynthia 30:36
Well, I always liked the idea - very much - of going into a part of biology that was really unknown. I really liked that. And when we first started working on developmental biology, when I was a postdoc, not much was known about it, but more was known. But then pretty quickly, it became pretty well understood, but aging was a real mystery. So that was very attractive to me. When I was in England, I was a postdoc with Sydney Brenner in England. And usually, you don't see old worms, you work on young worms. And the worms will overgrow the plates before they ever get old, so you never see them. During my postdoc, I was working with C. elegans; it wasn't very fertile. And worms reproduce by self fertilization. So you just put one worm on a plate; pretty soon you have a whole plate full of worms, like in no time at all. And I was working with a worm that wasn't very fertile. And so I came back, and I saw these old worms. And that was a real mind-bender for me, because, first of all, I didn't know worms got old, really. And second of all, I didn't know that if you looked at a worm, you could tell right away that it was old. So that was amazing. And the fact that worms have short lifespans meant that it's possible to do genetics. So in the back of my mind when I was a postdoc, I wanted to investigate this in more detail. And actually, it's really hard just practically to get someone to do the experiments. No one in my lab was interested in working on aging; they all thought I was crazy. They made fun of me. And so I would talk to rotation students. And I was the first author on the paper, because it was all done by rotation students; I had to keep them away from my lab members, because I was afraid the lab members would tell them that. They will apologize nowadays, the lab members, they say, “Yeah, we were pretty hard on you, Cynthia, but you were right.” I could have been wrong, you know, not all crazy ideas are right. But this one was. So there were five authors, and I was a professor. So I did almost all the experiments, and I wrote the paper. So I was first author. It says in the acknowledgments, of course, that the grants came to me and everything. But I think that's…you want to be studying something that generates resistance, where people say, “Oh, that will never work; that's a crazy idea.” Because if you think about it, and you're thinking logically, there's no reason that you can think of why this isn't a good idea. Like: all animals age, but at different rates, and evolution can change the rate, so maybe there are genes - that's a pretty good logical connection. So maybe I can find the genes. If you have that kind of logic, you can kind of hang your hat on that that can be kind of your north star. And if no one has a really good reason that makes logical sense, then it's good because it means you're studying something new. And if you're right, it will make a difference. So that's what you want to be doing.
Anthony 33:12
You know, a lot of our listeners are trainees, and I can't imagine a better piece of advice for them to hear that, truly. I want to thank you so much, Cynthia; I've followed your work since I first started med school. And it's such an honor to be able to sit and talk to you today.
Alex 33:27
Thank you, Cynthia. This is just an awesome conversation.
Cynthia 33:29
I hope so thank you so much.
Anthony 33:39
We usually take time at the end of each episode, in the spirit of regular in person meetups in Boston many years ago, to discuss a big problem, the nail and possible solutions, and the hammers, inspired by what we just heard. Alex, do you have a hammer or nail this week?
Alex 33:58
I have a nail. I don't know how to categorize it. Maybe it's a nail. I think it's a nail, I guess kind of stepping back through this whole season. We've heard about some experiments that are done on the lab bench. And then there's some experiments that really only can be done in human beings. And one of the great class of experiments in human beings that we can do is the pursuit of the biomarker, so some piece of information or hint in a living thing and a person that's indicative of disease.
Anthony 34:26
Ah, this is a great topic, you need to think about going back to the Framingham Heart Study, and the discovery of cholesterol as a biomarker for heart disease. You know, even in the conversation we just had with Cynthia Kenyon, one of the things that's really a holy grail in the aging field is trying to find biomarkers of aging. And earlier in the season, when we talked to Jay Bradner, we talked about liquid biopsy and biomarkers of cancer. I know this is something you've been thinking about. What's the biomarker you're looking for Alex?
Alex 34:55
Yeah, I'm really interested in a class of biomarker that I think has been understudied, and I think it's the biomarkers that we can find in our own breath. And this is actually one of the oldest diagnostic tools. So Hippocrates talked about diagnosing different diseases using the smell of his patients breath. So sweet breath was indicative of folks that had diabetes. So diabetes mellitus, the “mellitus” part of that formal name means honey, and that's a reference to the honeyed breath or honey taste of urine…
Anthony 35:28
I would much rather smell breath than taste urine, to be honest…
Alex 35:32
[Jovially] You know what, you just need to be more adventurous you know, you're a doctor, right, you should be able to just dive in and do what you need to do to help your patients. But these days, we do not use our noses or our tongues, largely to diagnose. But this is a very, very old tradition. And there's a paper in the 1970s by Linus Pauling, no less, assessing the number of metabolites or volatile organic compounds that are in breath. And he found that there were many hundreds and in fact, this field has been growing steadily. And progressively, the field is called Breath Analysis. And there's thousands of molecules in our breath, and these molecules come from the environment, alright, these molecules come from our own bodies that come from the food that we eat from all kinds of places. And breath is a really interesting place to go look for biomarkers, because it's a filtrate of blood. So the molecules that are in our breath, were in our blood. So these numbers kind of blew me away when I first heard about it - but there's about three and a half kilograms of blood that pass through your lungs every minute. And over the course of a day, that's five tons of blood that have touched air, and they're contacting 12 cubic metres of air. So this is a big room full of air that has exchanged molecules with your blood. And the molecules that come out of our breath are indicative of our disease state, there's been a ton of studies over many, many years that have showed certain molecules are indicative of certain diseases. But the thing is that the field is a bit fragmented. We haven't put together collectively as scientists any very clear high resolution map, or atlas, of the molecules that are in our breath and how they relate to our health. And so something that I've become really interested in figuring out if we can do, is can we build an atlas of human breath? We're trying to build a Human Cell Atlas. We've mapped the human genome, there's all kinds of maps and atlases that we're putting together as biologists that are critical for understanding our health. And breath is unmapped. And so I think that this is an incredible opportunity in front of us.
Anthony 37:41
So tell me a little bit more about what happens as I exhale. I've heard you say in the past that there are different phases of what comes out.
Alex 37:49
Yeah, yeah. So in one breath is kind of a whole city of different things. It kind of depends how you slice it. But I think of it in three phases, since… I've learned this from breath analysis researchers. So this is not something I've discovered, I'm just trying to relay my, my understanding. But the first phase is the really, really light gases like carbon dioxide, carbon monoxide, nitrogen, oxygen; those are very light gases, and they volatilize quickly. And then after that comes the volatile organic compounds, heavier molecules, but they're still light. So these are molecules that range between 50 and 350, or 400 Daltons so there's like, you know, maybe five to 20 different atoms in them. And some of them have a smell. So these are the molecules that are light enough to float in the air, but heavy enough to have some kind of content to them. And some of them we can detect with our own noses. And then the last phase is exhaled breath condensate. This is the stuff that actually can transmit viruses and bacteria. So not everything is just gas in our breath; some of its aerosol. Some of it's a little droplets of water that contain things in those droplets like proteins, DNA, RNA, viruses, bacteria. And inside of this whole breath, you've got everything, you've got all these different things that are coming out every single time that you exhale.
Anthony 39:10
So why haven't we explored the wealth of biomarkers available through breath? I mean, it's non invasive seems easier than checking blood. It's not as gross as stool or urine. Why aren't we paying more attention to it?
Alex 39:18
I think that there's a couple things that have changed over the last 10 years that made it possible to take a close look, whereas before it was not possible. The first thing is that the instruments to analyze the molecular content of our breath have gotten way, way better. Some of the metabolites in our breath that are important for understanding our health are super duper rare. But now we have these instruments, so they're fancy - either the size of a dishwasher or small car. They've got names like ‘gas chromatograph mass spectrometer,’ and they can detect very rare metabolites down to parts per trillion, sometimes below that. So the instruments are better now. And these instruments produce enormous amounts of data. That was a hard thing to deal with maybe 10 or 20 years. So now with the advent of sequencing, and this is stuff that you know, extremely well, Anthony, biologists now know how to deal with extremely large amounts of data. And the last two parts are kind of interesting, and not necessarily that technical, but we now know how to capture and store breath like we store blood. So that part of the breath phase that I mentioned, the exhaled breath condensate - that's liquid, you can breathe out for about 10 minutes and get a couple of millilitres of this fluid - you can put in an Eppendorf tube and freeze and keep for a generation or more. And then like the piece that is a bit more nuanced, is these large studies like the Framingham study that you mentioned - they showed us how to do large scale observational trials and do them cheaply. So these are all the things that have kind of happened over the last generation, and the UK Biobank particularly scaled up how we know how to do observational trials. But all these things come together. I mean, like, now is the time to actually take a close look.
Anthony 40:59
Well, one question I have is going back to the example of Framingham Heart Study, which I think is a great precedent for this effort. I mean, now we look at blood for lots and lots of things. But the early win was cholesterol and coronary artery disease, and kind of the association between those two was a new insight that changed the history of medicine. What's the class of diseases that you think would be uniquely suited to going after with breath?
Alex 41:22
That's a good question. There's a couple that have already been studied, where we know that there's a signature inside of breath for that disease. So tuberculosis: one is latent, actually can be examined in breath. There's a lot of work that's gone into that. Various kinds of cancers seem to have some signature in breath metabolically; I think it's kind of a per cancer specific story. But that seems to be amenable to diagnosis. And then, we also know: the state of your diet can be examined, or the state of your kind of readiness or fitness can be examined in breath. So for instance, as you are peaking in a workout, the level of isoprene, which is a small molecule that we can find in breath - the level of isolation goes up. And then as you rest that goes down. So it's still kind of the Wild West, though; we don't know what disease is going to be best examined, or best leveraged, or best understood through breath. But we do know that this is a source of biomarkers that's relatively untapped.
Anthony 42:24
Amazing. I have to say, I know this is something you're really passionate about, and I look forward to being able to hear more about it in the years to come as you work on it.
Alex 42:31
It'd be my pleasure. And we should do it together, man.
Anthony 42:33
Totally my friend.
Alex 42:41
So Anthony, this is our last episode in Season 3, all about the future. Can you tell me what your take on the future is now after all, that we've learned and all the amazing scientists that we've met?
Anthony 42:52
Yeah, I mean, so first, I'm reminded of the words of the great Yogi Berra, “I worry about making predictions, especially about the future.” Yeah, let me start there. And I think there's maybe kind of two classes of takeaways that I felt from this season, which is about the direction that science is heading in, and a lot of the topics that we have. And then the second is about some of the people and a few patterns or meta patterns that I saw as we talked to these luminaries this season. First, when I think about the trends of where science is going, what I perceive to be true, is that we're very much in the era of integrative sciences, where a lot of the biggest discoveries are happening at the interface of different fields, and bringing in new ideas from one field into another. You know, I think this is very clearly a strong trend that we saw in almost every episode, and every guest this season, where, you know, it was kind of different than - let’s say - the early golden age of biochemistry, when we were discovering DNA and RNA and the structure of proteins, where it was very much people with incredibly focused questions that relied on very pure scientific investigation. Here, I think what you're seeing a lot more of is people that actually are spanning interfaces and crossing disciplines to make insights at the interface of two fields. And then, you know, the second thing that I was really struck by in quite literally every guest that we met, and maybe this is not a new thing, it's always been there - But the degree to which people who are able to make breakthroughs have a bit of a contrarian streak. Almost every one of them had an idea that they were passionate about. And it sounded a little crazy. And a lot of people dismissed it because it was impossible, or somebody else had already tried and failed, or because it was ‘a bad idea.’ But each of them had the ability to persevere and power through it. And I think that was just very inspiring as a life lesson as well. So about you, my friend, what were your biggest takeaways from the season? And what do you think the future looks like?
Alex 45:00
Yeah, I mean, I would start also with a pithy quote about the future to hedge my ability to predict it. Alan Kay said, The best way to predict the future is to invent it. And I think we've gotten a chance to talk with a group of people that are doing exactly that. I'm also reminded by a phrase used by the father of venture capital, Arthur Rock, talking about intellectual book value, or ideas that are inherently valuable, and if put to practice will induce massive transformations. And I think we also got to hear a lot of ideas and people working on ideas that have exactly that property. So I agree with all the points that you made. And I'll try not to be redundant and just highlight some other things that that popped up. What I noticed is that a lot of times, the information that led to a breakthrough was available - and this kind of ties in with your contrarian streak that you mentioned - the information was just there. Cynthia Kenyon decided to look at worms longer than other people; Karl Deisseroth decided to go look at these opsin proteins that had been in molecular biology textbooks for I guess, decades. So the stories of Invention and Innovation often start on a foundation that's been there for quite some time. And I was just struck at how consistent this story was that people aren't making things up from whole cloth. They're not drawing down divine inspiration, but they're using all of the information and all of the resources that are available in ways that are surprising, or ways that aren't the current way of thinking about things. So that really resonated with me. I also heard a thread of the increasing importance of data and ‘just looking.’ A lot of these fields that we entered into and had conversations about are like ‘awash,’ or if you want to have a negative tone, ‘drowning in data,’ and kind of apropos of our discussion about maps and in building embeddings, and stuff like that, I think that there's an emerging need or desire to do on directed exploratory data analysis and have patterns and data emerge, and us as humans to kind of pick the best patterns, but do this with computers as partners, right? So to kind of have them be like ‘bicycles of the mind,’ as it were. It reminds me of this concept introduced by a science fiction writer actually named Stanislaw Lem, one of my favorite writers in a book that he wrote, which is nonfiction called SUMMA TECHNOLOGIAE. He talks about an information barrier that we might be up against, that we're generating so much data, we're writing so many papers that we can't possibly keep up. And I'm already feeling this in my work. I don't know about you, Anthony, but I can't read all the papers that are coming out. Yeah. And I think what I'm starting to hear the rumblings of is that we're moving up against this information barrier, where all the data is available, very little of it is used, and even less of it is used productively. And so that seems to be almost an oncoming crisis. But I view that more as an opportunity, right? So there's this oncoming opportunity to work even more closely with computers, to deepen our pattern recognition abilities, and to deepen the kind of science that we can do.
Anthony 48:08
Amazing. You know, I can't think of a better way to wrap up this season. I had so much fun with you this season, my friend.
Alex 48:14
I had so much fun with you as well. This was awesome. Let's do it again. Huge thanks to Professor Cynthia Kenyon. As you know by now, this is the last episode in Season 3. If there are topics you'd like us to cover in Season 4, please email them to theoryandpractice@gmail.com or tweet @GVteam. We'd love to hear from you.
Anthony 48:38
This is a GV podcast and a Blanchard House production. Our science producer was Hilary Guite. Executive producers were Rosie Pye and Duncan Barber, Toby Matimong mixed the programme with music by Dalo. I'm Anthony Philippakis.
Alex
I'm Alex Wiltschko. And this is Theory & Practice.